Magnetic Nanoparticles (MNPs) are particles of nanosized range (10−9 nm)(usually ,100 nm in size) with unique properties of magnetic targeting, biocompatibility, surface modification characteristics and superparamagnetic properties. The application of magnetism in medical science was first introduced in the 1950s for “magnetic hyperthermia therapy” (cancer cell death) leading to various MNPs’ syntheses including Superparamagnetic iron oxide NPs (SPIONs).
The unique features of SPIONs makes them multifunctional “theranostic systems” in various biomedical applications as diagnostics (e.g. immunoassays), movement (cell sorting), treatment (e.g. drug, protein and peptide delivery), and imaging (MRI contrast agents, magnetic nanoparticle imaging).
Preparation
The most common methods for the preparation of MNPs include coprecipitation, microemulsion, thermal decomposition, solvothermal, sonochemical, microwave assisted, chemical vapor deposition, combustion synthesis, carbon arc, spray and laser pyrolysis synthesis and polyol.
pH and ionic strength of the precipitation solution can directly affect the size of synthesized MNPs by the coprecipitation method; as there is an indirect correlation between pH and ionic strength and the size of the synthesized particles. pH and ionic strength also affect the electrostatic potential of the surface of MNPs and their consequent dispersion stability. Using the microemulsion method produces smaller and more uniform particles.
In the polyol method, fine metallic particles are synthesized by reducing their dissolved metallic salts and direct metal precipitation from solution containing a polyol. MNPs have also been synthesized by electrochemical deposition of metal on a cathode, produced by reduction of metal ions dissolved from the anode.
A group of Gram-negative prokaryotes, magnetotactic bacteria, have demonstrated an ability to synthesize fine iron oxide nanoparticles in the size range of 50–100 nm. These bacterial MNPs are covered with phospholipid layers rendering them biocompatible. Natural protein components (e.g. ferritin) have also been employed as nanoshells with a central core within which iron oxide nanoparticles 6–8 nm in size can be synthesized.
Structure
Iron oxide nanoparticles such as magnetite (Fe3O4) and its oxidized form maghemite (g-Fe2O3) are the most common MNPs. Highly magnetic materials such as cobalt and nickel are susceptible to oxidation and therefore toxic. Two generic types of magnetic nanoparticles have been used: iron oxide core with a polymer coating (Core-shell structure) and polymeric nanoparticles with incorporated iron oxide crystals (Embedded matrices).
Core-shell protection
A superparamagnetic core is surrounded by a protective outer shell layer, which mediates electrostatic interactions between particles and controls oxidation or prevents it. The outer layer can also be useful in biomedical applications by making the nanoparticles biocompatible and able to circulate long enough in the body to reach their target tissue. The outer shell can be made of surfactants, polymers, silica, precious metals, or oxides. Some nanoparticles are coated with a single outer shell layer, and other types can be made into multi-layered nanoparticles with two or more functions combined.
Embedded matrices
Another method used to prevent nanoparticle agglomeration is to embed them into a polymer, carbon, or silica matrix, or attaching them to the surfaces of larger particles. This strategy can also be considered adding a ‘magnetic pigment’ to a material. This prevents the irreversible binding of nanoparticles to each other, but it also limits their free mobility, which could be undesirable based on the application at hand.
Physicochemical characteristics
Since the early introduction of MNPs the major goal has been to improve their structure according to their application; shape, size, and surface properties. Optimizing these three factors will increase their concentration in general circulation, decrease nonspecific cellular interaction and adverse effects, increase target cell internalization and reduce administered dose.
- Shape
The shape of MNPs can affect their in-vivo biodistribution, clearance, biocompatibility and cell toxicity. Spherical magnetite and maghemite particles offer a uniform surface area for coating and conjugation of targeting ligands or therapeutic agents.
- Size
The size of MNPs plays a major role for their biomedical applications such as magnetic cell separation and purification and the enhanced permeability and retention effect, which causes concentration of the particles in target tumor tissue. Although MNPs of smaller than 2 nm are not recommended for biomedical application as they diffuse through cell membranes, damaging intracellular organelles and thus exhibiting potentially toxic effects.
The most common techniques for measuring the MNPs’ size are transmission electron microscopy, dynamic light scattering, and the Scherrer method using x-ray diffractograms. As only a small number of the particles prepared are of the desired size, it becomes necessary to carry out fractionation of magnetic fluids. Currently applied techniques are centrifugation, size exclusion chromatography, and field flow fractionation. While the first two techniques separate particles on the basis of their density or size, the latter technique utilizes the magnetic properties of MNPs for their efficient fractionation.
- Surface properties
The surface charge of MNPs is a very important and determining factor for their colloidal stability and bodily distribution affecting their target cell internalization, for instance positively charged MNPs can diffuse easily within negatively charged breast cancer cells. Surface charge of MNPs also plays a role in their dispersion stability which doesn’t allow them to agglomerate on storage. The cellular internalization of MNPs also depends on cell types, that is particles with a hydrophobic surface are easily engulfed by reticuloendothelial cell (opsonization) resulting in their rapid plasma clearance and low blood circulation time.
Properties
Superparamagnetism
SPIONs are small synthetic maghemite, magnetite, hematite and mixed oxides of iron with transition metal ions (such as copper, cobalt, nickel, and manganese) which show “superparamagnetism” phenomenon (although size-dependent and limited to MNPs of 10-20 nm); i.e. they become magnetized following the application of an external magnetic field (i.e. magnetic separator) and can be guided within their containing vessel (e.g. for protein purification) or to their in-vivo target tissue (e.g. anticancer targeted drug delivery) and do not possess this properties after removing the magnetic field which doesn’t result in agglomeration, thrombosis or clumping conditions.
Particle surface coating
Surface coating plays a role in the functionalization of the nanoparticles. MNPs that have undergone “surface coating” with hydrophilic polymers (e.g. polyethylene glycol: hydroxyl or amino functional groups) can avoid engulfment by the reticuloendothelial cells which in turn increases their blood residence time and therapeutic effect. Surface coating of MNPs with different functional groups and ligands imparts different surface characteristics, making them suitable for a wide variety of biomedical and other industrial applications.
It also minimizes the aggregation of the uncoated ones, protects the surface oxidation, improves surface conjugation for drugs and targeting ligands, minimizes nonspecific cell binding and thus low adverse effects. MNPs can be coated either during synthesis or adsorption after synthesis. Surface coating of MNPs however must not change the properties of favorable features of the uncoated MNPs.
Conclusion
Magnetic nanoparticles have recently been used as a multifunctional tool in different sectors and industry and look very promising for future applications as they offer the possibility of tunable composition, morphology, and size, which renders them unique in terms of properties and applications. The fabrication of tailor-made complex magnetic nanoparticle formulations in conjugation with active moieties, such as drugs, enzymes, and targeting ligands, has proven to exhibit a multitude of applications.
Related news
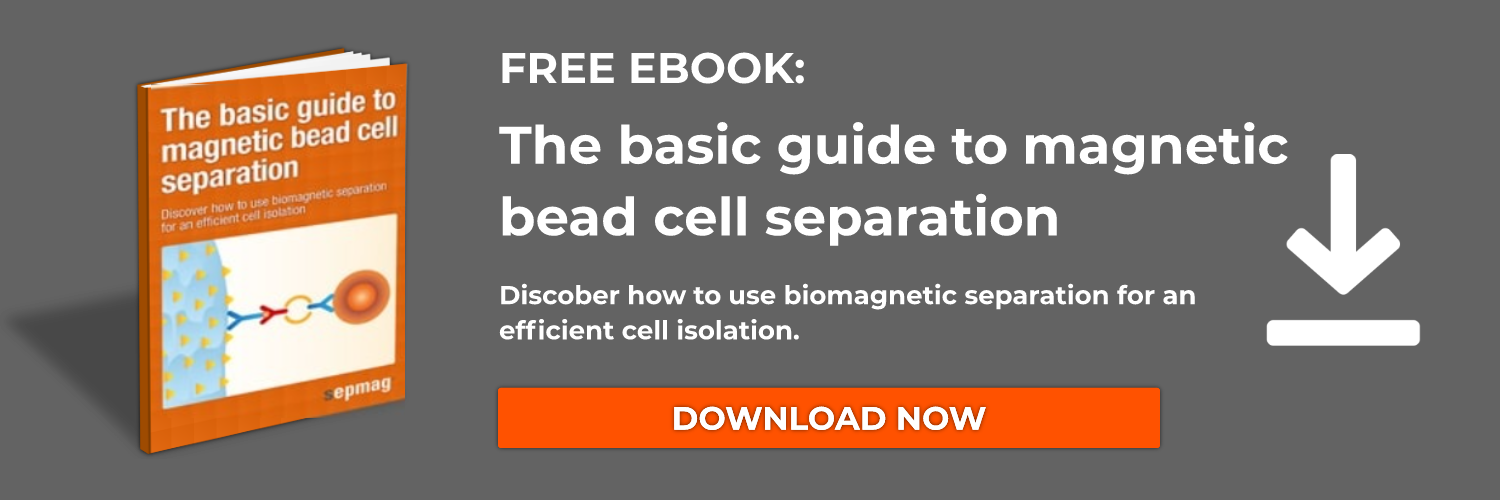